RUDY LAB
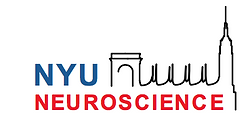

Morphological reconstructions of neurogliaform GABAergic interneurons identified using Id2 intersectional genetics
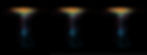
Superposed axonal reconstructions of the four interneuron populations found in mouse neocortical layer 1
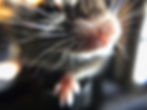
A mouse's whiskers serve as a primary component in its ability to sense its physical surroundings

Morphological reconstructions of neurogliaform GABAergic interneurons identified using Id2 intersectional genetics
DEPARTMENT OF NEUROSCIENCE & PHYSIOLOGY | NEUROSCIENCE INSTITUTE | NEW YORK UNIVERSITY SCHOOL OF MEDICINE
Organization and function of neocortical circuits
We are interested in how neuronal activity regulates behavior. Toward this goal, we study how brain function depends on the properties of individual neurons and the circuits they make with other neurons. Our main focus is on the cerebral cortex, the largest and most complex part of the mammalian brain. The computations that take place in this brain area are essential for perception, memory, motor control, and cognition. It is where acquired sensory information about the external world is integrated with past experience (memories) in order to predict and command appropriate behavior. We aim to understand how the basic properties of different types of cortical neurons – the building blocks of the cerebral cortex – and their connections contribute to behavior. We study the diversity of cortical neurons and the connectivity among different cell types, as well as the ion channels and other intrinsic factors that control their excitability, the dynamic properties of their synapses, and how these properties are regulated by neuromodulators. We then manipulate neuronal properties and cortical circuits and study how these manipulations affect function. We are currently emphasizing two areas of research: i) characterization of the circuits by which distinct GABAergic interneurons shape cortical information processing and ii) the mechanisms by which acetylcholine, a neuromodulator with key roles in the control of cognitive function, modulates cortical function.
To address these questions, we use a multidisciplinary approach that combines molecular, genetic, and anatomical techniques with electrophysiological, imaging, and optogenetic methods in vitro and in vivo, as well as behavioral methods. We hope to unravel cellular and circuit mechanisms that mediate cortical function and gain insight into how dysfunction of ion channels, cortical neurons, and neuromodulatory systems contributes to neurological and psychiatric disease.
Current Projects
Circuit mechanisms of top-down modulation of sensory processing and perception
Sensory perception depends on the integration of input provided by sensory organs, often referred to as “bottom-up” input and internal information, such as expectations, predictions, attention, emotional state, sensory-motor integration, and memories, often called “top-down” input. This project is focused on elucidating the mechanisms that mediate top-down modulation of sensory processing, leading to the generation of a percept appropriate for behavior.
Layer 1 of the neocortex, sometimes referred to as “enigmatic” or a “crowning mystery” is the main cortical target of cortico-cortical and subcortical glutamatergic and neuromodulatory projections that mediate top-down modulation. This layer contains the most distal, apical tufts of pyramidal cells in layers 2, 3 and 5. Input received by the dendritic tufts is integrated with sensory input received closer to the soma. Therefore, it is the circuits of L1 that mediate the integration of top-down and bottom up information. The impact of top-down projections on the distal dendrites of pyramidal cells is controlled by poorly understood inhibitory circuits that include the axons of SST-expressing Martinotti cells in layers 2, 3 and 5, the dendrites of several L2/3 GABAergic cells with prominent dendritic arbors in layer 1, and a resident population of L1 GABAergic cells, which was recently shown by our group to consist of at least four distinct interneuron sub-populations.
This project combines state-of-the art and innovative electrophysiological and imaging approaches in acute slices with in vivo optical and electrophysiological recording methods in head-restrained behaving mice. The goal is to discover the principles by which the various L1 inhibitory systems regulate the impact of top-down signals on pyramidal cells. Genetic manipulations are used to study how these circuits affect neural signals and performance in a whisker touch-dependent object localization task.
Mechanisms of cholinergic modulation of cortical function for sensory perception and learning
Acetylcholine (ACh) release and its associated actions on cortical networks have crucial roles in normal cognitive function and are implicated in human disease. Pharmacological observations in humans, and a large body of experimental evidence in animal models, strongly implicate ACh in arousal, attention, sensory gating and memory processes. This project focuses on the functional organization of the neurons that provide cholinergic projections to the cortex, as well as the cellular and circuit mechanisms by which ACh regulates specific cortical functions.
An increasing number of observations have challenged the classical notion that the cholinergic system implements a global, diffuse broadcast of modulatory signals across the entire neocortical mantle and have suggested that there is a large degree of specificity of the cortical cholinergic projection and a remarkable spatiotemporal precision in the actions of ACh on cortical networks. The majority of the cholinergic projections to the neocortex arise from neurons in the nucleus basalis and associated nuclei in the basal forebrain. While there is increasing evidence for the existence of specific functional streams of cortical cholinergic modulation, to date the lack of methods to target subpopulations of cholinergic neurons with specific areal or laminar projection patterns has limited investigation of such potential diversity and its functional implications. We hypothesize that cholinergic neurons with distinct innervation patterns are driven by different excitatory influences, resulting in different activity profiles during behavior. This project investigates the organization and diversity of cholinergic neurons projecting to the cortex by developing genetic strategies to tag and manipulate defined subpopulations of cholinergic neurons. The genetic models are used to investigate the inputs that drive the activity of distinct subpopulations of cholinergic neurons and to study their role in sensory perception and learning.